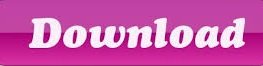
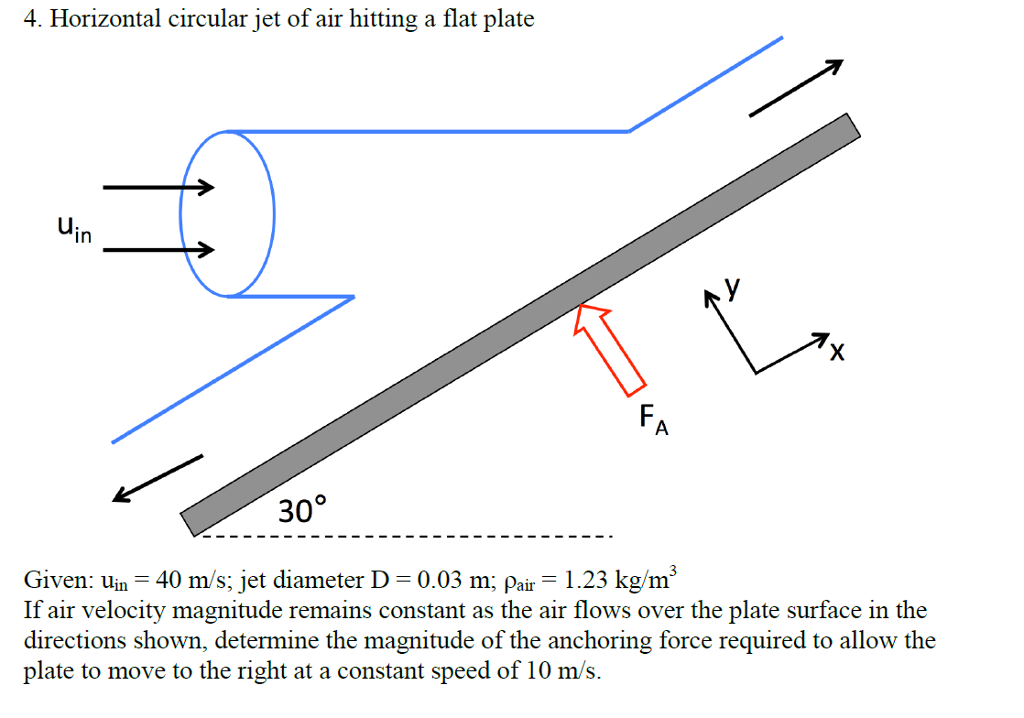
4SA (left), 3SA (middle), and 1SA (right) have four, three and one functional subunit(s) (colored), respectively. Probing SA variants with different valencies by AFM and ITC.Ī Schematic structure of SA constructs with different valencies. through fluid flow or rinsing steps.įigure 2. We expect our results to be highly relevant for force spectroscopy, and, in general, to improve assays where the SA-biotin bond is under load, e.g.
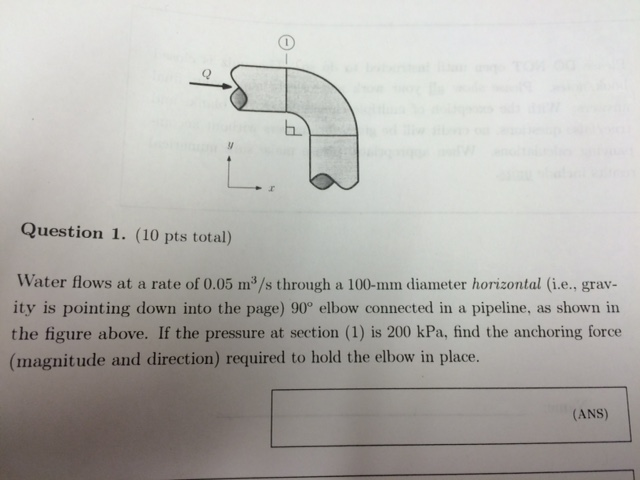
By using one well-defined geometry and a monovalent SA construct, a single-exponential lifetime is achieved. The different stabilities give rise to multi-exponential lifetime distributions for multivalent constructs. With an MT assay we assess the stability of the SA-biotin interaction under constant load and demonstrate large differences in the lifetime depending on the attachment geometry. Furthermore, we employ isothermal titration calorimetry (ITC) to directly measure the binding enthalpies of the different SA variants.
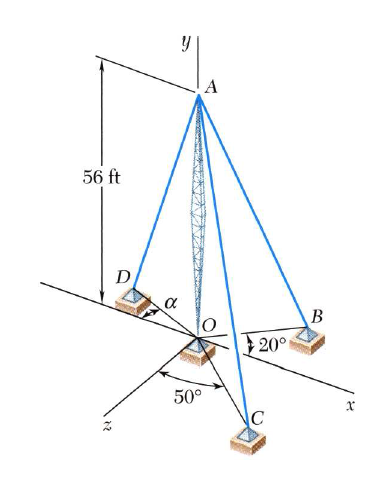
We use AFM imaging to verify the valencies by showing that only the competent subunits can bind biotin. Here, we employ engineered variants of SA with different defined valencies and a unique tethering point to restrict and control the number of possible force-loading geometries for SMFS measurements. However, the influence of the tethering geometry of SA on the lifetime of the SA-biotin interaction under constant forces is currently unknown. For some of the pulling directions, the SA subunit is deformed such that the energy barrier of the binding is decreased, causing lower biotin unbinding forces 24. Furthermore, if SA is non-specifically attached − as is the case in many commercially available SA-coated magnetic beads − a variety of attachment points combined with tetravalency results in an even larger range of possible force-loading geometries 20, 22.Ītomic force microscopy (AFM)-based constant speed SMFS experiments have recently shown that the force needed to unbind biotin from the SA binding pocket is strongly dependent on the force-loading direction 23, 24: Tethering SA by a single defined residue and pulling biotin out of one of the binding pockets results in different force-loading geometries, depending on which SA subunit the biotin has bound to. This ambiguity results in four different force-loading geometries for a given attachment of the SA tetramer ( Figure 1) 21. Despite its widespread use, SA’s tetravalency poses a problem, in particular in SMFS applications, since it is a priori ambiguous which of the four subunits biotin binds to. The long lifetime of the SA-biotin complex under external forces has enabled constant-force SMFS experiments lasting for hours and even up to weeks in magnetic tweezers (MT) 19, 20. It serves as a molecular handle to anchor molecules of interest and apply forces and torques to them 5, 11- 18. Owing to the specificity of the binding, as well as the robustness of the complex, the interaction has in particular become a popular tool in the context of single-molecule force spectroscopy (SMFS) assays 10. SA is stable over a wide range of conditions and easy to handle 1. Biotin can readily be covalently attached to nucleic acids 4, 5, 6, proteins 7, 8, or linker molecules 9. The non-covalent, high-affinity binding of the small molecule biotin to streptavidin (SA) is ubiquitously used in a variety of biological, chemical, biophysical and pharmaceutical applications 1, 2, 3.
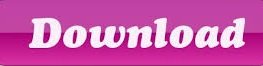